Should we strive for zero exposure? Researchers went underground for answers.
By the 1920s, Dr Marie Curie could barely see.
The two-time Nobel Prize winner had horrific cataracts caused by radiation exposure, and like many researchers of the day, she had no idea of the dangers.
“Perhaps radium has something to do with these troubles, but it cannot be affirmed with certainty,” Marie wrote in a letter to her sister Bronya in 1920.
Dr Curie would later die of aplastic anaemia, also due to radiation exposure. She was one of many researchers and workers to suffer due to the unknown effects of radiation, but she was definitely not the last.
Since their discovery, humans have tried to tame the X-rays, gamma rays and other radioactive particles which make us sick. We’ve used it for boundless energy from nuclear power stations, but deformed children, and poisoned thousands of kilometres of land with disasters like Chernobyl.
We’ve harnessed it for conflict, and as the war in Ukraine reminds us, there’s still 10,000 nuclear warheads around the world to this day.
Radiation – despite all the benefits of using it in medicine – can still be incredibly dangerous. But on the other end of the spectrum, there’s another problem: scientists aren’t sure what happens to humans (or any life at all) when the radiation is gone.?
“When you look at things that are harmful to life, we usually tend to look at extremes like extreme heat, extreme lack of heat, high pressure, low pressure,” says astrobiologist Dr Jennifer Wadsworth from the University of Lucerne, who specialises in radiation. “And with radiation, it should be no different. One should maybe ask the question ‘what happens when you take the radiation away?’”
Inside a castle, in a lab, in a mine
In a bid to answer this question, Dr Wadsworth headed down an active mine a kilometre underground in the northeast of England.
The lab her experiment was set up in was built for an entirely different purpose: to detect dark matter. The team of astrobiologists needed to be so far underground to avoid as much background radiation as possible, and dark matter detectors need similar cosmic quiet to catch any whiff of the elusive particles of dark matter.
“You’ve got a massive dark matter detector, staff and then our little slice of the lab where we built our castle,” she says.
In a lead box which the team called “the castle” they housed bacillus subtilis? and? E. coli? at a range of radiation levels, from 0.01 to 100 times the radiation levels the bacteria would normally be exposed to on the surface. They simulated this by placing amounts of caesium-137 right above the plate reader. It’s probably not a good idea to lick the caesium, Dr Wadsworth explains, but otherwise it’s safe to hold.
In their short experiment, the bacteria seemed completely unfazed about the amount of radiation, happily living their lives eating and replicating until the experiment ended. There was seemingly no difference from any of the radiation levels over the five or six days each experiment ran for.
But this is not the end of the story.
The team couldn’t get the radiation levels down to zero, just very low. And Dr Wadsworth was doing this experiment as an astrobiologist, looking at how bacteria and other simple organisms could survive in far flung places across the galaxy.
“With regard to space exploration and maybe life detection, some planets could be particularly well insulated from radiation. Maybe the rocks that [the planets] are built out of aren’t very radioactive. Maybe the organisms live deep underground, maybe they’re protected by water, ice,” she adds.
“It’s just an interesting thought experiment to try and understand how life might be on different planets.”
Instead, this experiment suggests a fascinating story of humans and how we adapt to background radiation. And it may shed light on one of the big controversies over how radiation affects lifeforms even at minimal, background levels.
With low levels of radiation being such an important part of medicine, it is surprising that we would know relatively little about how it affects humans’ large collection of complex cells.
But first, let’s clarify – no radiation at all is probably not a good idea for humans. RMIT medical radiation physicist Dr Pradip Deb thinks it would be impossible for us to live without.
“Light is also a radiation. Heat is a form of radiation. Everything we do, any source of electromagnetic waves is radiation and we cannot live without electromagnetic waves,” says Dr Deb.
“We cannot survive without radiation.”
What about ionising radiation?
Although microwaves, mobile phones and the dreaded 5G have all been demonised at times, the truth is that these are all low frequency radiation sources.
This means that they do not have enough energy to ionise atoms in our body.
Imagine just one ionising radiation particle is whizzing through your body. The particle will hit one of our atoms and push out one or more electrons from that atom, otherwise known as ionising the atom. Because electrons are so unstable, they generate indiscriminate chemical reactions like reactive oxygen species throughout the cell. Depending on where the cell is hit it can either damage parts of the cell, parts of the DNA, or both.
In this example, because there’s only a tiny amount of radiation, your cell machinery is likely to be able to repair the cell, fix the DNA break, or induce programmed cell death in the single cell to easily take care of the problem. Very occasionally during the repair process the DNA might repair incorrectly, causing a mutation in the DNA, which could lead to cancer down the road.
Nuclear power, CT scans, UV radiation from the sun, and even bananas do expose the participant to small amounts of ionising radiation. This type of radiation is either from high frequency electromagnetic waves (such as gamma or X-rays) or particles (beta, alpha or neutron particles).
However, it’s important to note that it’s not just radiation that causes these DNA mutations. Mutations can occur due to a mix up when copying genes, virus infection or exposures to mutagen chemicals. Plus, mutations are essential to evolution.
Even before human-made radiation came on the scene, we’ve long been exposed to lower levels of natural ionising radiation.
Most of this is due to radioactive gases radon and thoron, which are created when other elements decay in rocks and soils. The gases then rise out of the ground and into basements and ground floor dwellings, then the gas becomes trapped in buildings. The average radon exposure in a US home is 2.28 millisieverts (mSv), but in Australia it’s far less.
Our government’s Australian Radiation Protection and Nuclear Safety Agency (ARPANSA) has conducted a nationwide survey of radon in homes. The results showed that the average concentration of radon in Australian homes is low and about one quarter of the worldwide average.
“Consequently, there is little cause for concern that the health of the population is at undue risk from radon in homes,” the agency explains on its website.
“However, this survey did find that approximately one in a thousand homes may have high levels of radon. Generally, homes that are well ventilated, made of timber or built on stumps have lower radon levels compared to homes on concrete slabs with brick walls. However, the only way to be certain of radon levels in your home is to get it tested. Radon monitors are available for purchase from ARPANSA.”
Cosmic rays are also a source of natural radiation – although the atmosphere protects us from most of it, some of them can penetrate through giving us a dose of around 0.3 to 0.8mSv per year (depending on how far above sea level you are).
Comparing this to a whole-body CT scan of 10mSv or much higher in cancer therapy, the likelihood of this affecting humans in any way is minimal. But it illustrates that ionising radiation has always been something humans have been exposed to. Living on a planet where these DNA mutations occur has meant that our bodies are seemingly able to deal with these minimal levels of radiation without much trouble.
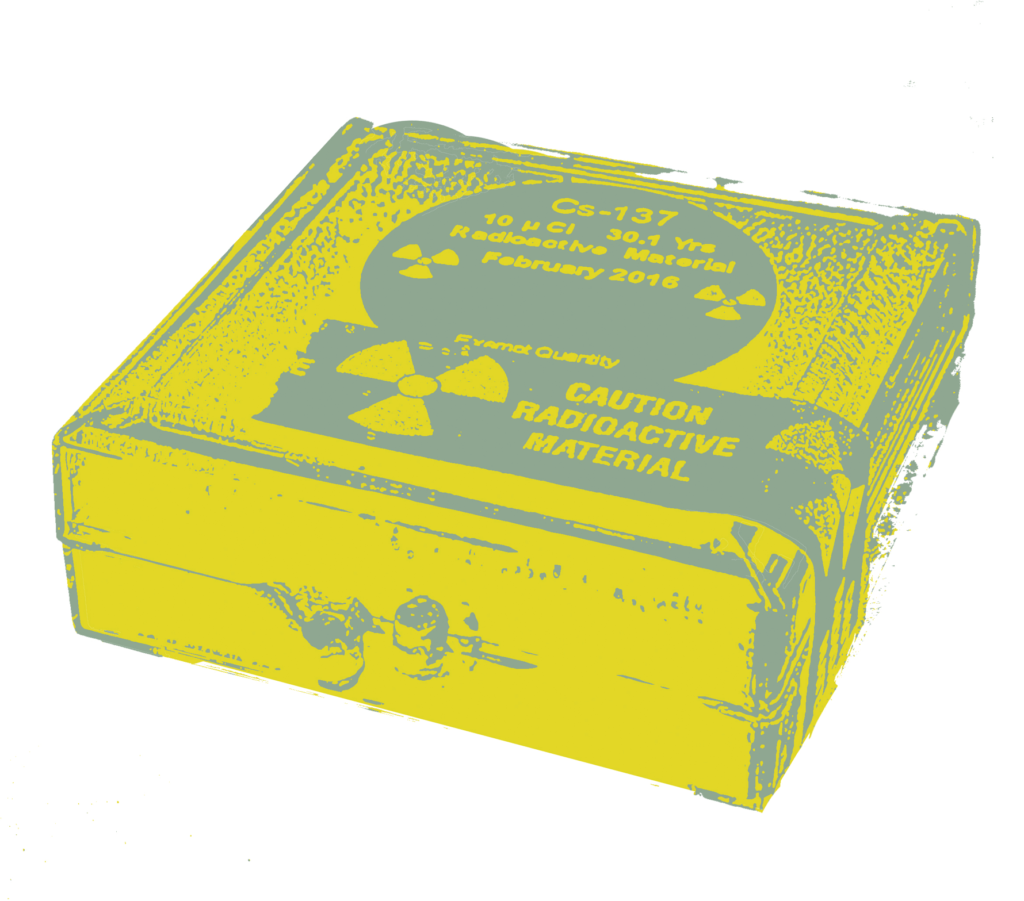
The linear no-threshold model
One of the reasons Dr Wadsworth undertook her research was to understand more about the effects of lower radiation doses.
One of the models used by most health agencies and government regulators to evaluate risk, known as the linear no-threshold (LNT) model, is highly controversial.
“LNT is not TNT, but differences in opinions sometimes appear explosive!” Professor John Boice, Jr., the former president of the National Council on Radiation Protection and Measurements, has been quoted saying.
The LNT model puts forward that any incremental additional dose of radiation, no matter how small, increases our risk of cancer, genetic mutations and other effects.
This model was designed looking at data from atomic bomb survivors and found that at 100mSv your cancer risk is 1.05 times baseline and goes up from there.
This holds true at high levels of radiation, but the accuracy of it at lower radiation levels is very hard to quantify. This is because while we have plenty of studies of high ionising radiation doses, it’s much harder to look at what happens at lower levels.
Shows like Chernobyl chronical what happens to humans when they are quickly exposed to a high amount of ionising radiation. At 4000mSv, there’s a 50% chance you’ll die from exposure within a month. 1000mSv can cause radiation sickness like nausea, vomiting and haemorrhaging. But a 2006 analysis of the health effects of low level radiation suggests even that large of a dose only increases your cancer risk from 22% to 27% over a lifetime.
Lower levels of radiation over a much longer timeframe become much more difficult to untangle.
The LNT is up against two competing theories. First is the threshold model, which posits that radiation below a certain level has zero effect on humans, and that radiation only becomes harmful when it exceeds a that threshold.
The second is called radiation hormesis. This hypothesis argues there is a level of radiation (within the region of natural background levels or just higher) which could actually be good for us.
Dr Wadsworth’s bacterial mine research hints that, at least in bacteria, that the threshold model could be right. However, she couldn’t eliminate radiation altogether, which would have provided more accurate results. She also suggests that leaving the experiment for longer – with more generations of bacteria – could lead to different results.
Of course, this might hold completely differently in humans. Much more research will need to be done in both model organisms and us before we can know for sure.
Is a little radiation really that bad?
Looking at these very low levels of radiation and what it does to us is an interesting thought problem, but it’s not the only issue. The LNT model also shows the unknowns about the dangers of slightly higher levels of radiation.
Dr Nina Stewart – a radiation oncologist at GenesisCare in Western Australia – describes learning about the LNT model in university, long before starting clinical practice.
The risk of the LNT model being true has meant that in the past decade there’s been a push for radiation oncologists like Stewart to ‘deescalate’ radiation when trying to treat cancers and expose patients to as little radiation as necessary.
“It’s something that we all keep in the back of our minds … particularly with young people,” she says.
“We’re reducing dose, we’re getting more accurate with our target verification, we’re using stereotactic radiotherapy.”
But it’s not just those unlucky enough to get cancer that are exposed to higher levels of radiation than the average population.
When you leave the relative safety of our atmosphere, the amount of ionising radiation you experience goes up dramatically from cosmic rays or solar particle events. This can be a problem for both astronauts, and even pilots and cabin crew as they fly through the thinner, higher levels of atmosphere.
“My husband’s a pilot,” says Dr Stewart, “He’ll often speak about colleagues that feel they have a higher incidence of cancer because of higher levels of ionising radiation when flying,”
There are radiation limits for both flight personnel and astronauts to make sure they’re limiting their exposure, but despite the potential risk, the science shows there’s less to worry about than you might imagine.
US aircrew have an average annual dose of over 3mSv – one of the largest of all US radiation-exposed jobs, according to the CDC. Astronauts on the other hand might end up with a whopping 300mSv after a year in space, according to NASA.
But so far at least, we haven’t been able to find an increase of cancer risk or mortality in those who regularly take to the skies, even astronauts.
“It needs to be monitored,” says Dr Deb. “But we haven’t found anyone who has got cancer because of flight.”
The citizens of Ramsar, a city in Northern Iran, live in a place with the highest levels of natural background radiation in the world, up to 131mSV a year in some buildings, according to the Journal of Radiation Protection. This is significantly higher than the 20mSv limit imposed on those that work in radiation, and higher even than the 100mSv amount that we have evidence for an increased risk of cancer.
On the contrary, researchers have found nothing of the sort. Although there’s only about 2000 people who live in the high radiation area of Ramsar – which makes it much more difficult to study large effects – studies have shown no differences between cancer rates, nor physical and mental disabilities, in this area of Ramsar and elsewhere, according to an article in the International Congress Series.
Dr Deb suggests that an interesting – if very impossible – experiment might be to take people from lower background radiation areas and leave them in Ramsar for a while to see what happens.
“That is definitely not allowed,” he quickly adds. “No ethics would approve that.”
This isn’t to say there aren’t cases where radiation exposure has increased risk. According to research in BMC Cancer, diagnostic medical radiation workers can end up with higher likelihood of getting cancer over their lifetime, although this percentage has gone down significantly since the early days of X-rays, subsequent research in Insights Imaging shows.
“I don’t worry too much about my background radiation exposure [while working],” says Dr Stewart.
“I think there’s a lot more in terms of our environmental and perhaps lifestyle factors now that are putting us at greater risk than background radiation, in terms of risk of malignancy.”
A complicated case
So far, there’s been minimal and sometimes conflicting evidence to show whether the threshold model, LNT or radiation hormesis is correct at low doses. The problem is untangling these small amounts of ionising radiation doses compared to lifestyle factors, genetics and all the other things that cause cancer in the human body.
“With these low levels of radiation, it would only be a long-term effect and that’s really difficult to quantify. [How would you tell that] this incident that happened in 1995 led to a cancer diagnose in 2010?” says Dr Jacinta Yap, a post doctorial researcher at the University of Melbourne specialising in particle therapy.
“Everything in the human body is really complicated.”
In World War I, Dr Curie spent her time funding, building and driving around portable X-ray machines to help men injured on the battlefield. These machines were lifesavers for thousands of solders – allowing doctors to see any bullets or shrapnel inside the body and accurately remove them.
But many of the X-ray workers who Dr Curie taught and ran the vans were overexposed to radiation and suffered devastating burns.
We’ve come a long way since this wild west of radiation exposure, but the push and pull of balancing radiation treatment verses minimising exposure remains.
“We know [the risk of radiation treatment] is very small, but if that risk does then lead to a second malignancy, it’s a huge, life-altering, and potentially lethal consequence,” says Dr Stewart.
With radiation treatment being one of the largest exposures most of will have to ionising radiation, the importance of understanding the effects of low dose radiation is vitally important.